Benefits of Geothermal Energy
Overview
When properly developed and managed, geothermal systems are a clean, abundant, and reliable source of renewable energy. Use of geothermal energy for electricity generation or for direct use conserves non-renewable and more polluting resources. Installed geothermal electricity generation capacity world-wide is equivalent to the combustion of nearly 30 million tonnes of coal or the output of about 10 nuclear plants.
Geothermal energy is effectively a renewable resource that does not consume any fuel or produce significant emissions. Although some geothermal fields have been degraded, none have ever been exhausted and sustainable development is possible. Geothermal energy also has the advantage over other renewables that it is independent of climatic variation.
Geothermal energy is a relatively low-cost and indigenous generation option that can contribute to New Zealand’s growing demand for electricity. It is uniquely reliable, with geothermal power stations typically achieving load factors of 95%, compared to typical load factors of 30 – 50% for hydro and wind power stations. The Wairakei power station has operated at a load factor of more than 90% for over 40 years with low operating costs. This inherent reliability makes geothermal generation a valuable component in a diverse electricity supply system such as New Zealand’s.
There are geothermal generation opportunities on either side of Auckland (i.e. Northland and the Taupo Volcanic Zone), the principal demand centre for electricity in the North Island. Proximity to Auckland, assuming sufficient transmission capacity, provides an efficient, low-cost electricity supply option. Geothermal fields are also commonly coincident with major forests and their energy-intensive processing industries, allowing symbiotic development of each resource.
An important aspect of recent investment in geothermal projects is the development of partnerships between power generators and Maori trusts. Maori commonly have the land access rights to geothermal fields, and geothermal projects are increasingly delivering economic benefits to local Iwi.
Tourism
Geothermal tourism has a long history in New Zealand. Te Arawa iwi oral histories recount early visitors near Rotorua and Taupo districts and missionaries captured interest in geothermal attractions with their vivid descriptions, drawings and paintings. The early tourists came to bath in thermal waters and to see the geysers, springs, mud pools, and the once visible giant Pink and White Terraces of Lake Rotomahana (destroyed in 1886 by the eruption of Mount Tarawera). International tourism followed and by the 1860s, the people of Tuhourangi (local iwi) were fully engaged in tourism.
Interest in natural features and in the healing qualities of geothermal fluid continued to grow and today it contributes significantly to the local and national economy, including growing investment and leadership by Māori. Experiences include visiting natural features, and learning about Maori cultural links and traditional uses of the resource. People enjoy bathing in natural geothermal waters throughout New Zealand, from Ngawha Springs in Northland to Westland in the south and ‘wellness’ tourism is also an area of potential growth, provided the resource is managed sustainably.
The Whakarewarewa valley within the Rotorua geothermal field is currently the most visited tourist area in New Zealand, including the attractions of Te Puia and Whakarewarewa Village.
Mineral Extraction
Near surface geothermal alteration and associated weathered volcanic rocks concentrate a number of industrial minerals. New Zealand mineral companies mine active and fossil geothermal systems for a variety of raw materials including: colloidal silica, clay (Halloysite and Kaolinite), pumice, zeolite, perlite, and native sulphur.
Geothermal fluids also carry potentially valuable minerals such as lithium (for batteries) and amorphous silica (for semiconductors). Over the years several parties have conducted experimental extraction trials but none to date have proven economic. Currently the GEO40 company operates a pilot plant at Ohaaki for extracting ultra-fine silica for export markets http://geo40.com
Geothermal Uses and Values
New Zealand’s geothermal resources have been used for many years. Geothermal areas are important to Māori, who use the heated waters for cooking, washing, bathing, warmth, preserving, ceremonial use and healing. Māori also use geothermal minerals as paints, wood preservatives and dyes. In recent years, Māori have become significant players in the geothermal space with several of the major operators owned by iwi. Through many Treaty of Waitangi settlements, many iwi have had land returned to them and their traditional relationships with the geothermal resource formally recognised by the Crown.
Geothermal fields also have significant landscape, ecological, amenity, scientific and conservation value.
Other key uses include tourism, mineral extraction, direct heat use and electricity generation.
For more information on uses of geothermal energy, including New Zealand case studies –visit GNS Science’s Earth Energy webpages.
- 220ºC
Steam turbine and binary electricity or process steam - 140-220ºC
Drying, process heat, binary electrical plant - 70-140ºC
Space and water heating, drying - 30-70ºC
Thermoculture, bathing
Direct Use
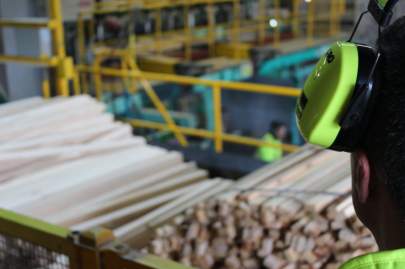
Direct use involves using geothermal heat directly (without a heat pump or power plant) for such purposes as heating buildings, industrial processes, domestic heating, greenhouses, aquaculture, public baths and pools. Direct use can use high and moderate to low temperature geothermal resources. Existing developments have generally been on an individual basis rather than capturing the benefits of combined use for district heating or industrial parks.
There is a wide range of direct uses in New Zealand for which consumer energy totals about 8 PJ. The largest geothermal direct user in the world is the Norske Skog Tasman pulp and paper mill at Kawerau. With an installed capacity of 210 MWt and an annual energy use of 7.5PJ, the plant uses geothermal fluids to generate clean process steam for paper drying, a source of heat in evaporators, timber drying and increasingly for electricity generation
Very low temperature resources can also be exploited for heat applications using ground-source heat pumps to provide space heating and cooling. This is a practical technology that can be used almost everywhere in New Zealand where some groundwater occurs and offers significant opportunities for electricity conservation; however, it cannot be used for electricity generation. For more on ground source heat pumps.
Development Potential
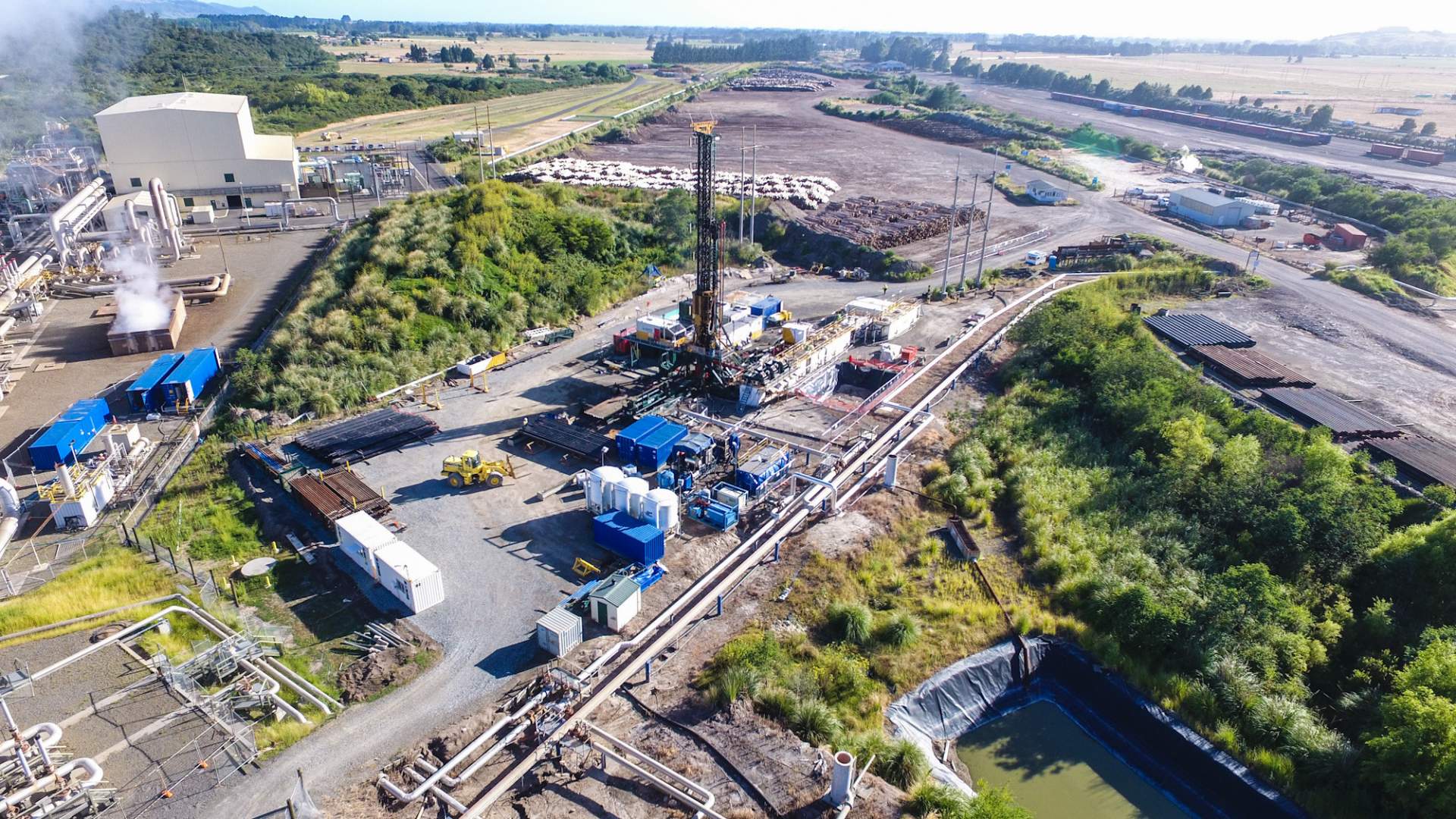
The information on this page is now out of date. The NZGA will be updating this page with the latest potential for geothermal development estimate.
Geothermal energy has the potential to make a significant contribution to New Zealand’s energy requirements, either through electricity generation or, with even higher levels of efficiency, as a direct source of heat. However, because geothermal steam cannot be transported for more than a few tens of kilometres, the opportunities for direct use are limited and large-scale electricity generation is the most attractive option.
New Zealand has substantial geothermal resources that are accessible and untapped. A comprehensive assessment of New Zealand’s high temperature geothermal resources is that by Lawless (2002), the total resource is estimated as equivalent to a about 3600 MWe of electrical generation, using only existing technology. Almost 1,000 MWe is currently installed, so existing development represents about 30 % of the total high temperature resource.
Emissions
Conventional geothermal systems occur naturally, due to deep heat sources such as magma chambers, which most often occur near plate boundaries where tectonics has induced melting of the Earth’s crust. The fluid in most geothermal systems is groundwater which is heated near this deep heat source and then moves upwards closer to the surface, a heat transfer process called convection. Geothermal systems are therefore dynamic systems, the size and shape of which depends on the depth and temperature of the heat source and the permeability structure of the shallower rocks through which the convecting fluid moves.
The chemical content of geothermal fluid depends on the original composition of the groundwater, any inputs of fluids from the magma chamber (or deeper), the composition of the rocks through which the fluid travels, and the pressure and temperature (which affect the rate of fluid-rock interaction). Geothermal fluid contains CO2, methane and hydrogen sulphide, and in the natural state (pre-development) these are discharged through obvious natural surface features such as fumaroles and bubbling pools, and less obviously as a flux through the soil.
After development of a geothermal system for power generation, during operation of the power plant some of the geothermal gases (CO2 and others) can become separated from the geothermal fluid as a result of changes in temperature and pressure. The gases that have become separated are non-condensable and are released to the atmosphere as a part of the power generation process.
It is useful to consider emissions for electricity generation in terms of an “emissions factor” (also called “emissions intensity” or “carbon intensity”) of grams CO2-equivalent per kilo-watt hour gCO2(eq)/kWh, which enables comparison to electricity from other energy sources. The measure “grams CO2-equivalent” is a useful way to combine the CO2 and methane into one number – it is the mass of actual CO2 plus an equivalent mass of CO2 to represent the methane. As a greenhouse gas, methane has 25 times more impact than CO2 and so the mass of methane times 25 gives the equivalent mass of CO2.
The emissions factors for geothermal power stations in New Zealand for the calendar year 2018 are given in the table and figures below. These are the emissions of CO2(eq) released from the geothermal fluid during operation of the plant. The median emissions factor for 2018 is 62 gCO2(eq)/kWh and this is a standard measure of the central tendency of this kind of dataset with outliers. The use of the median (and other percentiles) is the same approach as used by the IPCC in the 2011 Special Report on Renewable Energy Sources and Climate Change Mitigation. Another useful statistic is the weighted average of 76 gCO2(eq)/kWh, which is weighted using the total energy generated from each plant, thus accounting for the fact that not all plants are the same size and hence their individual numbers for emission factor do not carry the same weight.
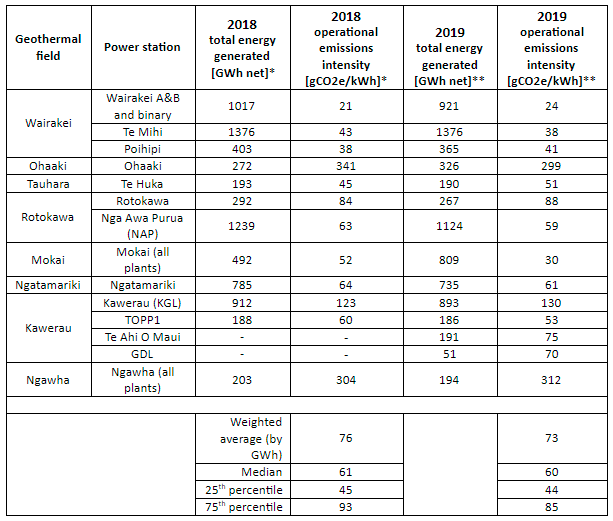
*Source: McLean, K. and Richardson, I. (2019): “Greenhouse gas emissions from New Zealand geothermal power generation in context”. Proceedings, 41st New Zealand Geothermal Workshop, Auckland, New Zealand, 25-27 November 2019***.
** Source: McLean, K., Richardson, I., Quinao, J., Clark, T. and Owens, L. (2020): “Greenhouse gas emissions from New Zealand geothermal: power generation and industrial direct use”. Proceedings, 42nd New Zealand Geothermal Workshop, Waitangi, New Zealand, 24-26 November 2020.
*** Geothermal conference papers available in IGA database.
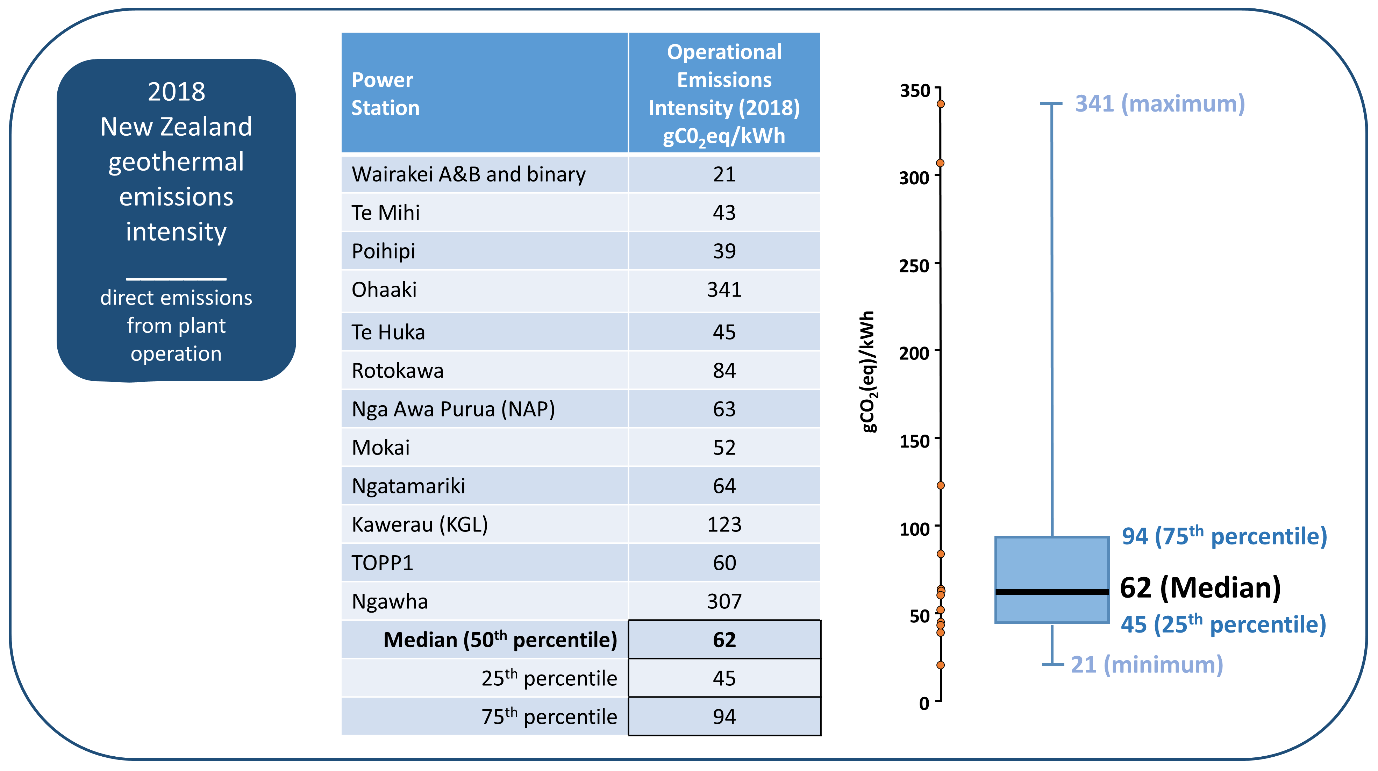
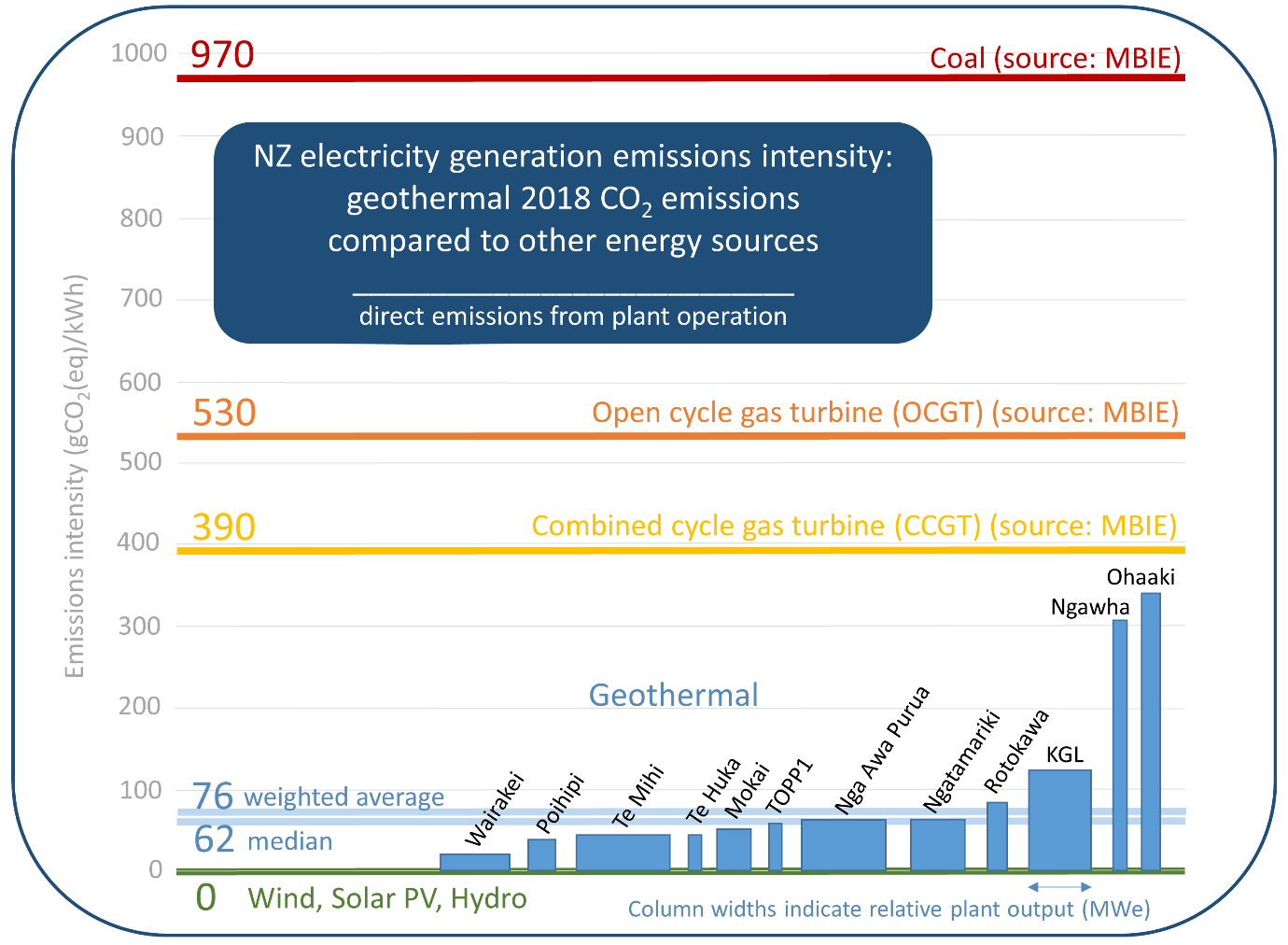
For comparison, the emissions factors from other renewable energy sources during operation are:
- Hydro: > 0 gCO2(eq)/kWh (some methane is emitted from decomposition of organic material in the reservoir, though this is hard to quantify)For comparison, the emissions factors from other renewable energy sources during operation are:
- Solar photovoltaic (PV): 0 gCO2(eq)/kWh (no emissions from sunlight)
- Wind: 0 gCO2(eq)/kWh (no emissions from wind)
And emissions factors from fossil fuel plants from fuel combustion during operation are (national average NZ, source MBIE):
- Coal: 970 gCO2(eq)/kWh
- Natural gas (open-cycle gas turbine): 530 gCO2(eq)/kWh
- Natural gas (combined-cycle gas turbine): 390 gCO2(eq)/kWh
All the emissions factors discussed so far are for operation of the plant only. For a true comparison between the different energy sources, the full life-cycle of the plant needs to be considered. A life-cycle assessment (LCA) includes carbon emissions related to: materials/construction, operation, and decommissioning. An international review of LCAs for all energy sources is available in the 2011 IPCC Special Report on Renewable Energy Sources and Climate Change Mitigation (see figure and table below).
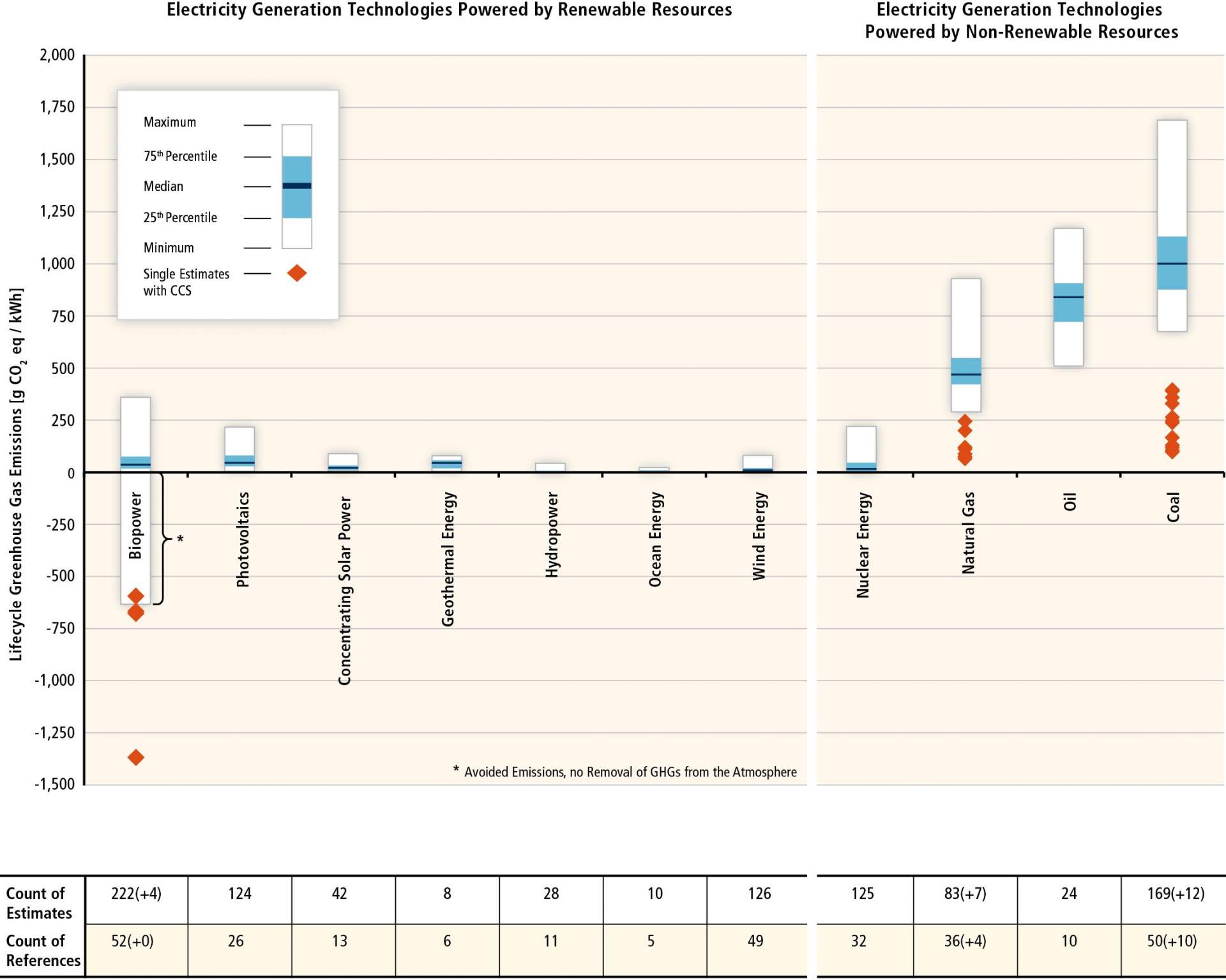
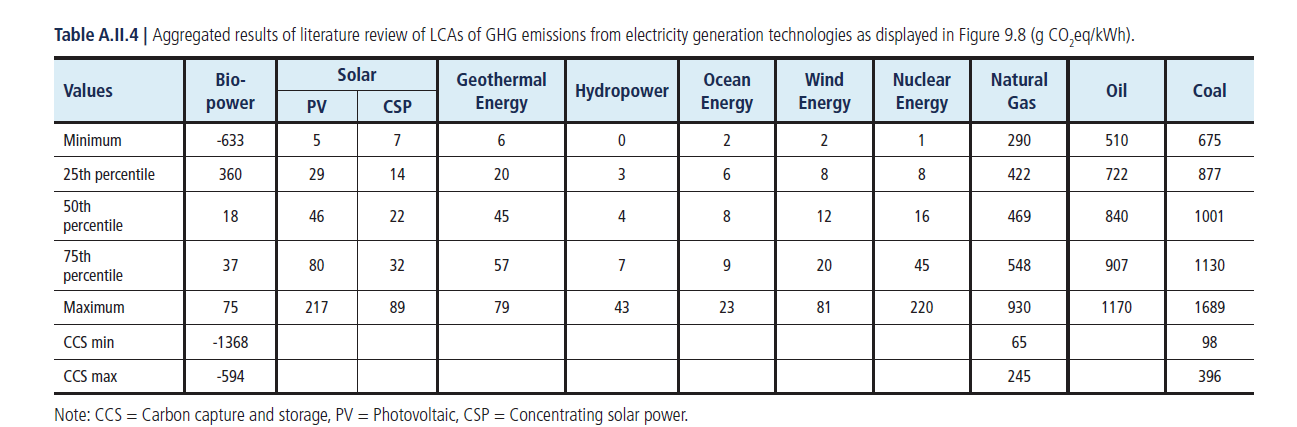
(Table A.II.4 from IPCC (2011): Special report: Renewable Energy Sources and Climate Change Mitigation)
For all the energy types currently present in NZ, median LCA CO2(eq) emissions factors from the IPCC report are as follows:
- Coal: 1001 gCO2(eq)/kWh (high certainty – 169 data points)
- Natural gas: 469 gCO2(eq)/kWh (high certainty – 83 data points)
- Solar PV: 46 gCO2(eq)/kWh (high certainty – 124 data points)
- Geothermal: 45 gCO2(eq)/kWh (uncertain – only 8 data points)
- Wind: 12 gCO2(eq)/kWh (high certainty – 126 data points)
- Hydro: 4 gCO2(eq)/kWh (medium certainty – 28 data points)
The IPCC life-cycle (LCA) median emissions factor for geothermal is 45 gCO2(eq)/kWh, which seems low when the median NZ emissions in 2018 were 62 gCO2(eq)/kWh from operation only. This may be due to the limited number of geothermal LCAs – only 8 data points met the criteria to be included in the IPCC review. All the other energy types had many more LCAs and are likely to be more representative.
Although the true median geothermal life-cycle emissions factor will likely be higher, it is still useful to consider these life-cycle numbers, as it can be seen that:
- All energy types emit CO2(eq), there is no zero-carbon energy.
- All the renewable energy types present in NZ (hydro, wind, solar PV and geothermal) have emissions factors at least one order of magnitude lower than the fossil fuel plants (gas and coal).
Geothermal emissions intensities are relatively complex, and change over time. The intensity decreases due to field degassing, and can increase or decrease to some degree due to operational changes to the steamfield or plant, as shown schematically in the figure below. Additionally, there are two important factors which offset some of the CO2(eq) emissions from geothermal electricity generation, which are not usually accounted for:
- Geothermal plants have the benefit of producing large volumes of hot water. This is often just reinjected back into the reservoir, however the hot water can be used (and is being used) as process heat for various industries, which would otherwise burn fossil fuels to obtain that heat.
- Geothermal systems in their natural state emit CO2 and methane from natural surface features, such as fumaroles, bubbling pools, and flux through the soil. Development of geothermal power stations has often resulted in a decline in surface CO2 and methane emissions, although this is very difficult to quantify.
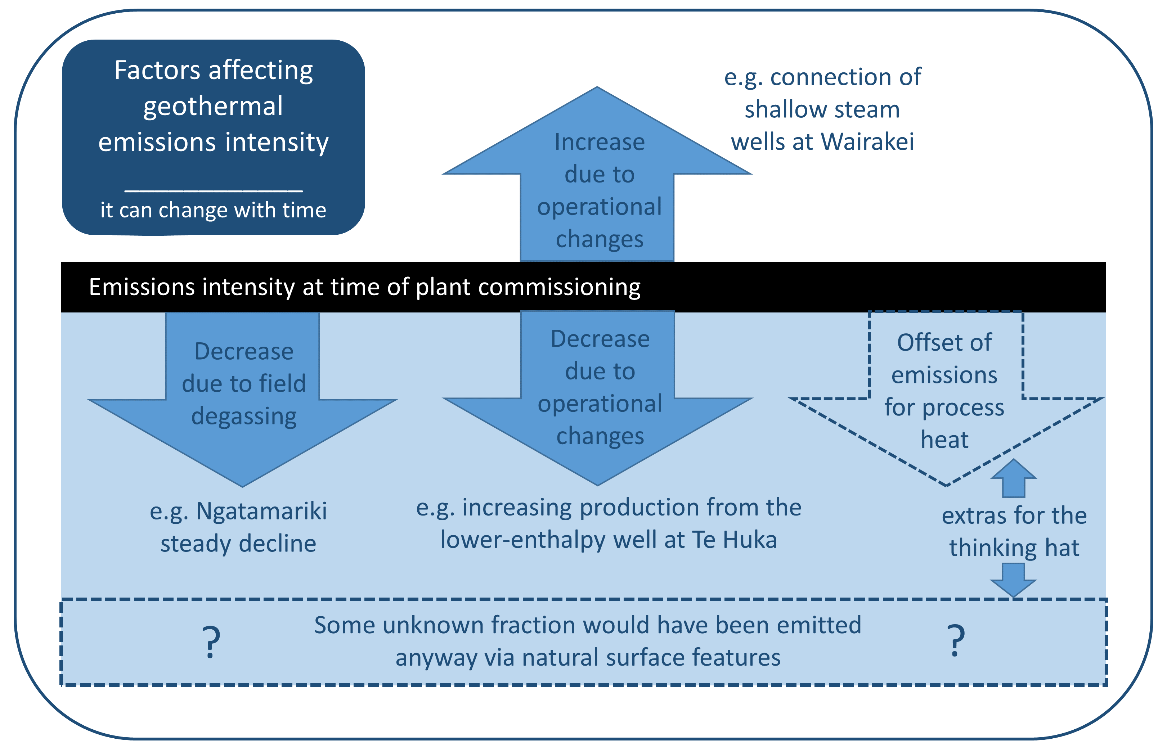
Geothermal Fluid Use and Emissions Trading Requirements
- Application – Electricity Generation and Industrial Heat
The New Zealand Emissions Trading Scheme applies to using geothermal fluid for generating electricity or industrial heat, where the emissions of carbon dioxide-equivalent (CO2-e) exceed 4,000 tonnes from a given installation per annum. - Legislative Requirements
The Climate Change Response Act 2002 (update as at 8 Dec 2009) requires industries to register, to set up holding accounts, to gather data, to monitor emissions, to provide regular data returns for prescribed periods at specified times. Payment is according to default emissions factors for a given facility as specified in the Climate Change (Stationary Energy and Industrial Processes) Regulations 2009 unless an application for a unique emissions factor is made and approved under the Climate Change (Unique Emissions Factors) Regulations 2009.
Geothermal facilities supplying geothermal fluid for generating electricity or industrial heat are subject to the Climate Change (Stationary Energy and Industrial Processes) Regulations 2009. These regulations consider fluid supply as either geothermal steam (Schedule 2, Table 6, Part A) or geothermal fluid (Schedule 2, Table 6, Part B). Prescribed or default emission factors are defined in Schedule 2, Table 6 of the regulations for these two fluid types. The measured annual fluid production is multiplied by the prescribed emissions factor to derive the reportable annual emissions from a given facility.
There is an option for the prescribed emissions factor to be substituted with a unique emissions factor. The methodology to develop a unique emissions factor for a geothermal facility is covered in the Climate Change (Unique Emissions Factors) Regulations 2009, clauses 14 to 17. Aspects of determination of unique emissions factors covered are in a letter on the Climate Change Act – Geothermal Sampling Procedures dated 23 September 2010 from GNS Science to the New Zealand Geothermal Association. This letter identifies appropriate sampling methods that comply with the legislative requirements.
Other Commentary and Information
A number of companies have analysed their processes, determined that it is cost effective to make an application for a unique emissions factor and have subsequently applied for and been granted a unique geothermal emissions factor.
The carbon emissions scheme effectively taxes industries for their emissions. For the geothermal industry, which has comparatively low carbon emissions, this increases their economic performance with respect to other higher emitting generators.
For more on the emissions trading scheme visit the NZ Government climate change web site
Links
Publications
- Practical methods of minimizing or mitigating environmental effects from integrated geothermal developments; recent examples from New Zealand
Chris Bromley
www.researchgate.net - Houghton, B.F. 1989: Inventory of New Zealand Geothermal Fields and Features. Geological Society of NZ
- B.F. Houghton 1982. Geyserland: A Guide to the Volcanoes and Geothermal Areas of Rotorua. Geological Society of New Zealand Guidebook N. 4.
- B.F. Houghton, E.F. Llyod and R.F. Keam 1980: The Preservation of Hydrothermal System Features of Scientific and Other Interest – A Report to the Geological Society of New Zealand.
- Parliamentary Commissioner for the Environment 2003. Electricity, energy and the environment. Part A making the connections.